Research
DIELECTRIC BARRIER DISCHARGE (DBD) - NOVEL ATOMIZERS FOR TRACE ELEMENT ANALYSIS
Contact person: Jan Kratzer
Introduction
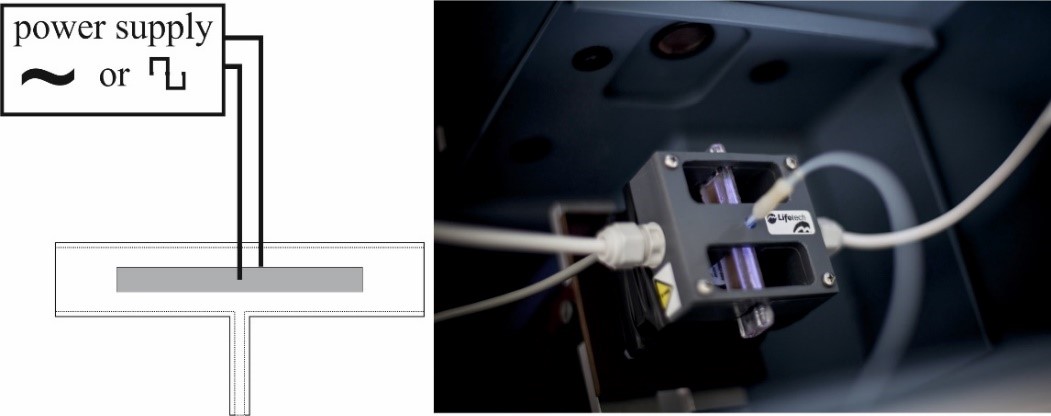
Atomization of hydride forming elements in dielectric barrier discharge (DBD) plasma atomizers with subsequent AAS detection is studied in our laboratory since 2013. Approaches to on-line atomization as well as in-situ preconcentration of analytes in the DBD atomizers are explored. Moreover, effort is made to further develop the design of DBD atomizer as well as the construction of its power supply source in order to achive the best performance. Mechanistic studies are indivisible part of our research since we believe that the best sensitivity and robustness of DBD atomizers cannot be reached without understanding the fundamental processes behind hydride atomization in the plasma.
Optimization of on-line atomization
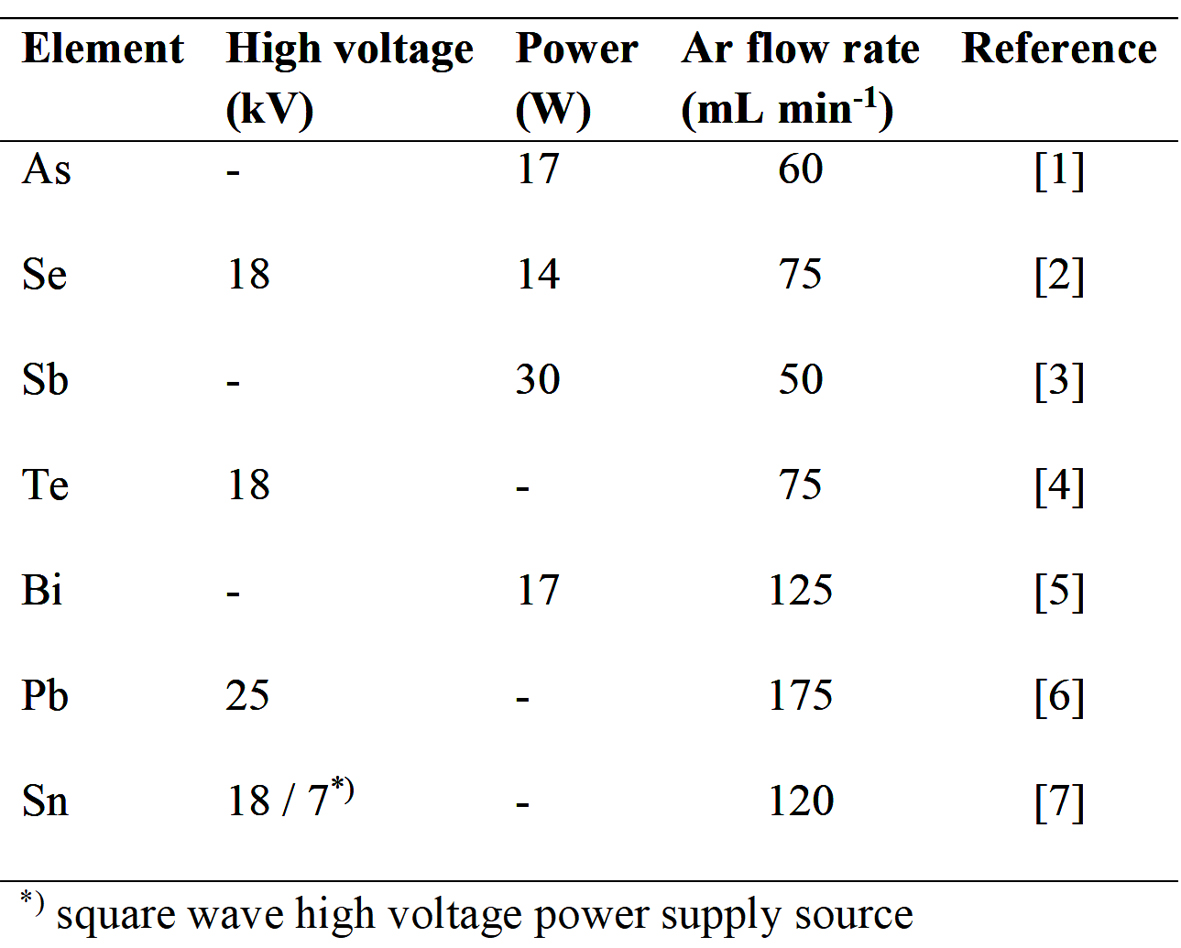
Atomization conditions of seven hydride forming elements in the DBD atomizer have been optimized so far. The results are summarized in Table 1. Although Ar was found as the best discharge gas for all the elements investigated, its optimum flow rate as well as the high voltage or power applied differ significantly among the elements. As a consequence, DBD atomization conditions have to be optimized carefully and individually for each element. The differences in optimum atomization conditions among the analytes are much more pronounced in DBD compared to those in externally heated quartz tube atomizers (QTA) where atomization temperature around 900 °C is applied regardless of the element and carrier gas flow rate oscillates around 75 mL min-1 Ar.
Analytical figures of merit
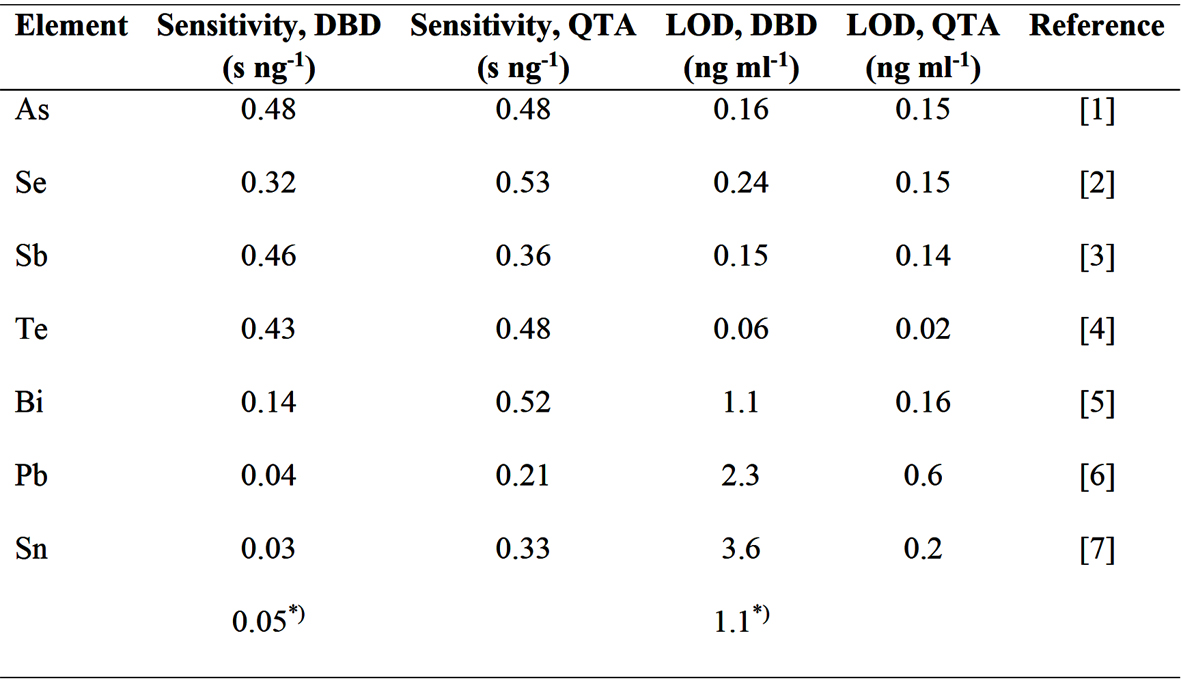
The performance of DBD atomizer under optimum conditions has always been compared to a QTA as a reference. Interestingly, the hydride forming elements investigated so far can be divided into two groups: As, Se, Sb and Te represent elements which hydrides can be easily and efficiently atomized in DBD providing high sensitivity and low LODs while atomization of Sn, Bi, and Pb hydrides is challenging reaching significantly worse sensitivity and impaired LODs (see Table 2). Moreover, sensitivity in QTA differs at maximum by a factor of 2.5 (Table 2). On the contrary, the difference in sensitivity among the elements reaches a factor of 16 in the DBD (Table 2). Effort is made to understand the hydride atomization in DBD and, based on this knowledge, to improve further DBD´s performance.
DBD atomizer design and power supply source construction
A planar (plane-parallel) DBD atomizer supplied by a sinusoidal AC power supply source (28 kHz) is typically used. The DBD electrodes are made of copper metal strips that are glued to the outer surface of the quartz walls of the DBD cell which serve as the dielectric barrier. If not stated otherwise, results presented in Tables 1-3 have been reached in this experimental arrangement.
However, employing sinusoidal power supply sources, the plasma current usually occurs as several peaks during a half discharge period which ratios and positions in time are not well defined. As a consequence, the energy delivered to the plasma is spread over these discharge events. For that reason a power supply source with a square wave high voltage modulation (28 kHz) was tested. The plasma current occurs at the rising and falling edge of the square wave voltage producing thus only one current peak in a half discharge period in that arrangement resulting in a temporally stable plasma. All the energy delivered to the plasma is focused into one discharge event. Sensitivity of Sn determination increased by a factor of 2.5 when the sinusoidal generator was replaced by a square-wave one [7]. On the contrary, the sensitivity of As determination was the same regardless of the power supply source employed [8].
It is advantageous to couple DBD designs with sputtered electrodes to the square wave generators since the losses of power are less pronounced compared to the DBD designs with glued electrodes [9].
Another feature typical for DBD atomizers of volatile compounds is the fact they are much more sensitive to the presence of aerosol droplets and water vapor, both co-generated together with analyte hydride. Their amount is dictated by generation conditions of analyte hydride, especially acid (HCl) and reductant (NaBH4) concentrations and their mutual ratio. More vigorous reaction leads to increased aerosol production. Since the power delivered to the DBD is much lower compared to QTA, significant power drainage from the plasma occurs resulting also in plasma instabilities in presence of aerosol and water vapor. To avoid this, special „dryer“ cartridges with various fillings should be employed. A dryer packed with solid NaOH improved signal of Sn in a DBD atomizer 3-times [7] while the same device increased signal of As only by 15% [1]. Applicability of the dryers is related to the physico-chemical properties of analytes, thus, there is no universal dryer applicable to all elements. For illustration, NaOH based dryers work well for As and Sn hydrides, but retain Se and Te hydrides completely.
In-situ preconcentration in DBD
Efficient preconcentration of analyte hydrides directly in the DBD atomizer prior to AAS detection was found feasible. The trapping and volatilization processes are controlled only by presence and absence of O2 in the discharge gas, respectively.
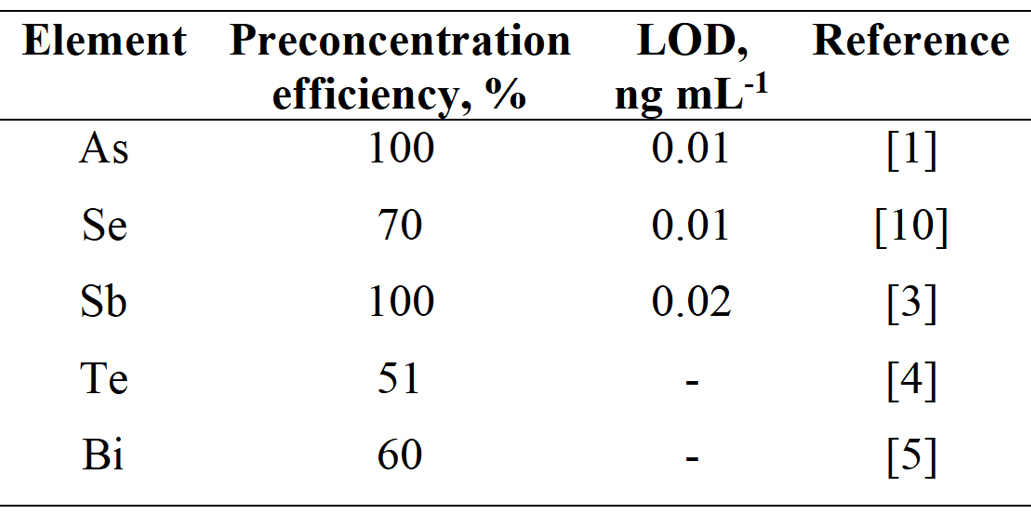
This makes the procedure simple and operator friendly. Addition of low O2 flow rates (~ a few mL min-1) to the discharge gas (ca 100 mL min-1 Ar) results in quantitative analyte retention. After a given preconcentration period, O2 flow is switched off, while the DBD discharge as well as the hydride generation of blank is kept running. Trapped analyte species are released by the Ar discharge that contains H2 evolved from hydride generation of blank. Five elements have been investigated so far (see Table 3).
LOD can be improved by an order of magnitude with 300 s preconcentration period compared to the mode without preconcentration. Mechanism of in situ trapping of hydride forming elements was in detail studied for Se only. Quantitative Se trapping in a narrow spot in the central region of the DBD optical arm was confirmed by a 75Se radioactive indicator while incomplete volatilization was found responsible for 70% overall preconcentration efficiency. Also in case of Te and Bi the incomplete volatilization is suspected to be responsible for overall preconcentration efficiency lower than 100%
Investigation of the atomization mechanisms
A variety of spectroscopic techniques including ICP-MS, radioactive indicators, direct analysis in real time (DART) coupled to Orbitrap-MS, spatio- and temporally resolved optical emission spectrometry (OES) as well as laser induced fluorescence (LIF) and selected ion flow tube MS (SIFT-MS) has been employed to investigate the atomization of hydride forming elements in DBD atomizers, often owing to our international and inland collaborations. Significant differences in behavior among the analytes were found.
Strong interaction of Bi with the inner surface of the DBD atomizer was confirmed with more than 90% of Bi deposited in the optical arm [11]. This fraction was only around 30% for Se indicating longer life-time of Se free atoms [12]. Temporally and spatially resolved OES measurements have shown that AsH3 is atomized and excited in the plane-parallel DBD atomizer across the whole discharge channel which explains its good sensitivity in AAS measurements [8]. Analogous OES measurements have revealed that the major part of Sn is atomized close to the walls of the atomizer. Thus, the probability of the decay of analyte atoms by deposition at the glass surface of the DBD atomizer increases [13].
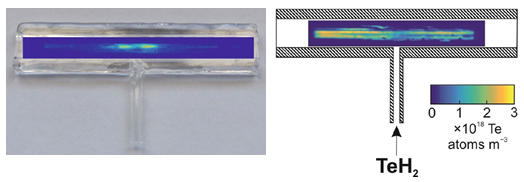
A mechanistic study by LIF has been performed to visualize the spatial distribution of free Pb atoms in the DBD atomizer as well as to quantify plumbane atomization efficiency [6]. Free Pb atoms were present only in the central region of the DBD atomizer and plumbane atomization efficiency was quantified only to 23 ± 7% [6]. On the contrary, the same LIF measurements have identified free Te atoms all over the length of the optical tube of the DBD and TeH2 atomization efficiency was quantified as high as 100 ± 7% [4] indicating clearly great differences in DBD ability to atomize hydrides of various elements.
The results of the mechanistic studies are in accord with the behavior of individual analytes observed in AAS measurements giving explanation for high sensitivity reached for As, Se and Te on one side and much lower sensitivity reached for Bi, Sn and Pb on the other (compare with Table 1). For details see the references including our review article on DBD principles and applications [14].
References
- 1. NOVÁK P., DĚDINA J., KRATZER J.: Preconcentration and Atomization of Arsane in a Dielectric Barrier Discharge with Detection by Atomic Absorption Spectrometry. Anal. Chem. 88 (2016), 6064-6070.
- 2. DUBEN O., BOUŠEK J., DĚDINA J., KRATZER J.: Dielectric barrier discharge plasma atomizer for hydride generation atomic absorption spectrometry - Performance evaluation for selenium. Spectrochim. Acta Part B 111 (2015), 57-63.
- 3. ZURYNKOVÁ, P., DĚDINA J., KRATZER, J.: Trace determination of antimony by hydride generation atomic absorption spectrometry with analyte preconcentration/atomization in a dielectric barrier discharge (DBD) atomizer. Anal. Chim. Acta, 1010 (2018), 11–29.
- 4. BUFKOVÁ K., MUSIL S., KRATZER J., DVOŘÁK P., MRKVIČKOVÁ M., VORÁČ J., DĚDINA J.: Generation of tellurium hydride and its atomization in a dielectric barrier discharge for atomic absorption spectrometry, Spectrochimica Acta Part B, 171 (2020), 105947.
- 5. KRATZER J., BOUŠEK J., STURGEON R., MESTER Z., DĚDINA J.: Determination of Bismuth by Dielectric Barrier Discharge Atomic Absorption Spectrometry Coupled with Hydride Generation: Method Optimization and Evaluation of Analytical Performance. Anal. Chem. 86 (2014), 9620-9625.
- 6. ALBRECHT M., MRKVIČKOVÁ M., SVOBODA M., HRANÍČEK J., VORÁČ J., DVOŘÁK P., DĚDINA J KRATZER J., Atomization of lead hydride in a dielectric barrier discharge atomizer: Optimized for atomic absorption spectrometry and studied by laser induced fluorescence. Spectrochim. Acta Part B, 166 (2020), 105819.
- 7. JUHÁSZOVÁ L., BURHENN S., SAGAPOVA L., FRANZKE J., DĚDINA J., KRATZER J.: Hydride generation atomic absorption spectrometry with a dielectric barrier discharge atomizer: method optimization and evaluation of analytical performance for tin. Spectrochim. Acta Part B, 158 (2019), 105630.
- 8. BURHENN S., KRATZER J., KLUTE F. D., DĚDINA J., FRANZKE J.: Atomization of arsenic hydride in a planar dielectric barrier discharge: Behavior of As atoms studied by temporally and spatially resolved optical emission spectrometry, Spectrochim. Acta Part B 152 (2019), 68-73.
- 9. STRAKA M., BURHENN S., MARSCHNER, K., BRANDT S., MARGGRAF U., DĚDINA J., FRANZKE J., KRATZER J.: Novel designs of dielectric barrier discharge atomizers for atomic spectrometry. Spectrochim. Acta Part B 146 (2018), 69-76.
- 10. KRATZER J., MUSIL S., DĚDINA J.: Feasibility of in-situ trapping of selenium hydride in a DBD atomizer for ultrasensitive Se determination by atomic absorption spectrometry studied with 75Se radioactive indicator. J. Anal. Atom. Spectrom. 34 (2019), 193-202.
- 11. KRATZER J., ZELINA O., SVOBODA M., STURGEON R., MESTER Z., DĚDINA J.: Atomization of Bismuthane in a Dielectric Barrier Discharge: A Mechanistic Study. Anal. Chem. 88 (2016), 1804-1811.
- 12. KRATZER J., MUSIL S., MARSCHNER, K., SVOBODA M., MATOUŠEK T., MESTER Z., STURGEON R., DĚDINA J.: Behavior of selenium hydride in heated quartz tube and dielectric barrier discharge atomizers. Anal. Chim. Acta 1028 (2018), 11-21.
- 13. BURHENN S., KRATZER J., DĚDINA J., FRANZKE J.: Influences of voltage shape and discharge gas on the temporally and spatially resolved emission characteristics of tin in a planar dielectric barrier discharge. Spectrochim. Acta Part B, 161 (2019), 105695.
- 14. BRANDT S., SCHÜTZ A., KLUTE F. D., KRATZER J., FRANZKE J.: Dielectric barrier discharges applied for optical spectrometry. Spectrochim. Acta Part B 123 (2016), 6-32.