Research
CHEMICAL GENERATION OF VOLATILE SPECIES – FOCUSED ON NEW ANALYTES
Analytical atomic spectrometry methods serve for extremely sensitive determination of elements. These methods include in particular atomic absorption spectrometry (AAS), atomic fluorescence spectrometry (AFS) and inductively coupled plasma (ICP) either with optical emission (OES) or mass spectrometry (MS). The techniques of sample introduction to all of the above-mentioned methods are very important. The most common approach is nebulization of liquid samples. This approach brings very low introduction efficiency since only 1-5% of analyte typically reaches an atomization or ionization source of the spectrometer while the rest remains in the waste. Employing volatile species generation (VSG), some elements can be converted to volatile compounds (species) prior to their spectrometric detection. The introduction of the analyte into the detector in the gaseous phase has several advantages. Firstly, the analyte is separated from the sample matrix which reduces risk of interferences. Secondly, much higher introduction and transport efficiency of the gaseous analyte to the detector can be achieved which results in enhanced sensitivity and thus lower limits of detection (LOD). And finally, extremely mild atomization/ionization conditions are sufficient for the analyte in the form of volatile species. However, the number of elements which can be converted to analytically useful volatile species is limited and there is no universal approach applicable for broad range of elements.
The oldest, most widespread and well established approach to conversion of elements to its volatile species is chemical generation of hydrides (HG). Acidified sample is mixed in the generator with NaBH4 (or KBH4) to form volatile hydrides of elements of IV.-VI. main group of a periodic table, i.e. As, Sb, Bi, Se, Sn, Pb, Te and Ge. Hg can be generated in the form of free atoms (cold atomic vapors). All of these are commonly generated for routine analysis. The structure of volatile species of Zn and Cd, generated also by this approach, has not been clearly identified yet but it is assumed that unstable hydrides and/or atomic vapors are formed.
Due to the above-mentioned principal advantages of VSG, there have been attempts in many laboratories to extend chemical VSG to the whole periodic table or at least to more transition metals. A successful chemical VSG using NaBH4 reaction has been described for 16 transition metals (namely Ag, Au, Co, Cr, Fe, Ir, Mn, Mo, Ni, Os, Pd, Pt, Rh, Ru, Ti and Sc) so far but only few analytical applications have been reported. The main drawback lies in low generation efficiencies which typically reach maximum of single units or tens of percent. The other problem is the nature of those generated volatile species which has not been conclusively proven yet as well as the mechanism of VSG has not been explained.
Since volatile species generated by this chemical approach are usually very unstable, with serious transport losses being observed in the gas phase, it is evident that a different reaction scenario should be applied to generate pure and more stable gaseous species of transition elements. Photochemical VSG provides a promising alternative to chemical approach and has been applicable to a broader range of elements (see paragraph Photochemical generation of volatile species – exploring alternative routes to generation of volatile species).
OUR RESEARCH ACTIVITIES IN THIS FIELD:
Our laboratory developed a special type of generator (Fig. 1) that fitted well for chemical VSG of transition metals [1]. Suitable reaction conditions were found and efficient VSG of Ag was achieved with reaction modifiers (surfactants) [2] and permanent modification of the generator with palladium [3], partly done in co-operation with National Research Council in Canada (NRC). This generator (Fig. 1) was further developed and used for a study of a mechanism of VSG of Ag [4,5], Au [6], Cu [7] and Pd [8].
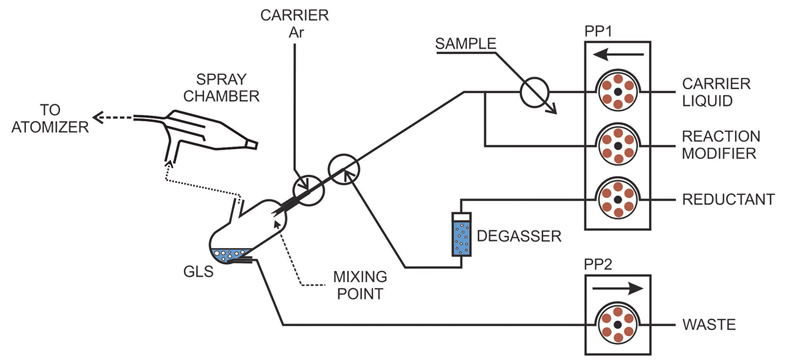
We attempted identification of the generated species using the instruments for determination of aerosol size distribution and transmission electron microscopy. The achieved results suggest that in the case of chemical VSG of Ag, Au and Cu enhanced only by the presence of surfactants, the generated species are not truly volatile but metal nanoparticles (Fig. 2) transported together with co-generated fine aerosol to the detector [4-7]. The latest results for Cu [7] further suggested that the species were probably even smaller than in nanorange, i.e. contained between single to tens of metal atoms. In the case of Pd, enhanced significantly by the presence of diethyldithiocarbamate (DDTC), the volatile chelate of Pd was clearly identified as volatile species by direct analysis in real time mass spectrometry (DART-MS) [8].
The key characteristic to evaluate the state of optimization of VSG is the VSG efficiency that is defined as the fraction of the analyte converted to a volatile species, released from the liquid phase and then transported to the atomizer or plasma for the detection. A separate review article was published on this topic, summarizing various approaches of determination of generation efficiency of several transition metals and dealing with their pros and cons [9]. Several approaches were also employed for determination of generation efficiencies at our laboratory.
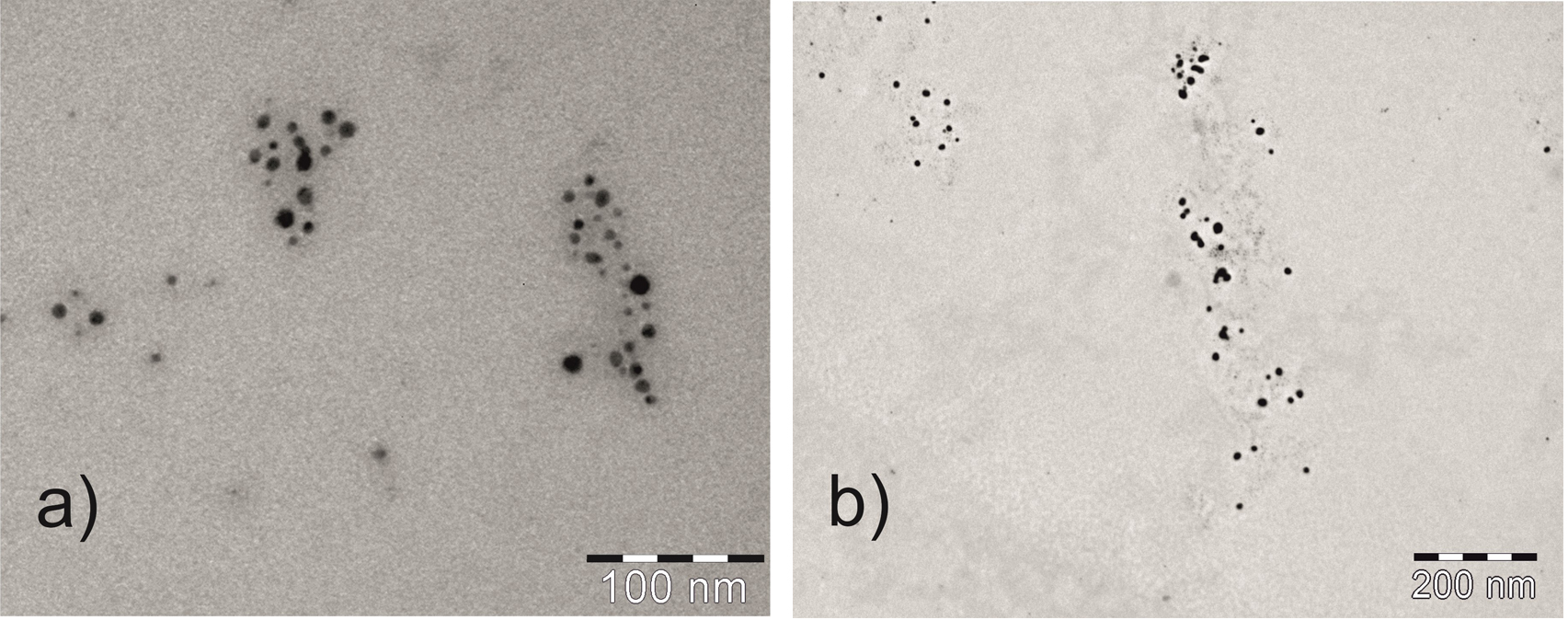
The most accurate way was demonstrated to be a trapping of the volatile species from the gas phase on a suitable filter with subsequent determination of its amount relative to that introduced to the generator. A very feasible way was the application of radiotracers of investigated elements (111Ag, 198,199Au). Another option was a comparison with direct nebulization ICP-MS. At chosen optimal conditions of VSG, we achieved VSG efficiencies of 8% for Ag [4], 12% for Au [6], 31-32% for Cu [7] and 16-22% for Pd [8]. It was also found that volatile species of Ag, Cu and Pd were transported in the gas phase with great transport losses. After modification of the quartz tube atomizer design, when the inlet arm was directly connected to the outlet from the gas-liquid separator and heated to 300 °C, VSG efficiency increased to 33% for Ag [5].
Preconcentration of volatile species from the gaseous phase prior their detection is an elegant way to decrease limits of detection (LOD) of spectrometric detectors, especially AAS. The most common approach is in situ trapping of volatile species in graphite furnaces. We developed a procedure for in atomizer preconcentration of Ag in a quartz tube atomizer, similarly as described in paragraph Preconcentration of volatile species for ultratrace and speciation analysis. The principle of this methodology lies in trapping of analyte in the optical tube using oxygen rich atmosphere while efficient release is carried out after switching to hydrogen rich atmosphere in the optical tube. A special modular atomizer with exchangeable optical tube was designed and more than 90% collection efficiency was achieved for Ag improving LOD approximately ten times [10]. This preconcentration methodology and analytical performance was further developed using the optical tube made of sapphire [11,12], with substantial increase of the optical tube lifetime which was only around 100 cycles for quartz optical tubes.
The atomization of Au volatile species in various quartz atomizers was also examined in detail. It was postulated that volatile species of Au were converted to AuH at elevated temperatures which was subsequently atomized by the hydrogen radicals [13].
RELATED PAPERS:
- [1] MATOUŠEK T., DĚDINA J., VOBECKÝ M.: Continuous flow chemical vapour generation of silver for atomic absorption spectroscopy using tetrahydroborate (III) reduction - system performance and assessment of the efficiency using instrumental neutron activation analysis. Journal of Analytical Atomic Spectrometry, 17 (2002), 52–56.
- [2] MATOUŠEK T., STURGEON R. E.: Surfactant assisted chemical vapour generation of silver for AAS and ICP-OES: A mechanistic study. Journal of Analytical Atomic Spectrometry, 18 (2003), 487–494.
- [3] MATOUŠEK T., STURGEON R. E.: Chemical vapour generation of silver: reduced palladium as permanent reaction modifier for enhanced performance. Journal of Analytical Atomic Spectrometry, 19 (2004), 1014–1016.
- [4] MUSIL S., KRATZER J., VOBECKÝ M., HOVORKA J., BENADA O., MATOUŠEK T.: Chemical vapor generation of silver for atomic absorption spectrometry with the multiatomizer: radiotracer efficiency study and characterization of silver species. Spectrochimica Acta Part B: Atomic Spectroscopy, 64 (2009), 1240–1247.
- [5] MUSIL S., KRATZER J., VOBECKÝ M., BENADA O., MATOUŠEK, T.: Silver chemical vapor generation for atomic absorption spectrometry: minimization of transport losses, interferences and application to water analysis. Journal of Analytical Atomic Spectrometry, 25 (2010), 1618–1626.
- [6] ARSLAN Y., MATOUŠEK T., KRATZER J., MUSIL S., BENADA O., VOBECKÝ M., ATAMAN O. Y., DĚDINA J.: Gold volatile compound generation: optimization, efficiency and characterization of the generated form. Journal of Analytical Atomic Spectrometry, 26 (2011), 828–837.
- [7] ŠOUKAL J., BENADA O., MATOUŠEK T., DĚDINA J., MUSIL S.: Chemical generation of volatile species of copper – Optimization, efficiency and investigation of volatile species nature. Analytica Chimica Acta, 977 (2017), 10–19.
- [8] VYHNANOVSKÝ J., KRATZER J., BENADA O., MATOUŠEK T., MESTER Z., STURGEON R. E., DĚDINA J., MUSIL S.: Diethyldithiocarbamate enhanced chemical generation of volatile palladium species, their characterization by AAS, ICP-MS, TEM and DART-MS and proposed mechanism of action. Analytica Chimica Acta, 1005 (2018), 16–26.
- [9] MATOUŠEK T.: The efficiency of chemical vapour generation of transition and noble metals. Analytical and Bioanalytical Chemistry, 388 (2007), 763–767
- [10] MUSIL S., KRATZER J., VOBECKÝ M., MATOUŠEK, T.: In situ collection of volatile silver species in a new modular quartz tube atomizer for atomic absorption spectrometry. Journal of Analytical Atomic Spectrometry, 27 (2012), 1382–1390.
- [11] MUSIL S., DĚDINA J.: A sapphire tube atomizer for on-line atomization and in situ collection of bismuthine for atomic absorption spectrometry. Journal of Analytical Atomic Spectrometry, 28 (2013), 593–600.
- [12] MUSIL S., MATOUŠEK T., DĚDINA J.: Sapphire: a better material for atomization and in situ collection of silver volatile species for atomic absorption spectrometry. Spectrochimica Acta Part B: Atomic Spectroscopy, 108 (2015) 61–67.
- [13] ARSLAN Y., MUSIL S., MATOUŠEK T., KRATZER J., DĚDINA J.: Gold volatile species atomization and preconcentration in quartz devices for atomic absorption spectrometry Spectrochimica Acta Part B: Atomic Spectroscopy, 103–104 (2015) 155–163.