Research
COMMON ATOMIZERS OF VOLATILE SPECIES IN AAS AND AFS
Contact person: Jiří Dědina
Introduction
One of general targets of our research is to develop analytical procedures for trace elemental and speciation analysis by atomic absorption spectroscopy (AAS) and atomic fluorescence spectroscopy (AFS) based on generation of volatile species (VS). The final step of the whole procedure of element determination or of speciation analysis based on generation of VS is atomization. Disregarding the preconcentration approaches based on in-atomizer collection treated in paragraph Preconcentration of volatile species and atmospheric plasma atomizers employing dielectric barrier discharge (DBD) treated in paragraph Dielectric barrier discharge atomizers, there are two types of atomizes of VS: (i) quartz tubes (QTA) for AAS, (ii) miniature flame devices typically employed for AFS but useful also for AAS.
(i) QTAs are T-tubes with the horizontal arm (optical tube) aligned in the optical path of the AAS spectrometer. The central arm of the T-tube serves for delivery of hydride carried by a flow of purge gas. The most popular type of QTA is conventional QTA. Its optical tube is externally heated to a temperature between 700 °C and 1100 °C. At least a small fraction of hydrogen accompanied by traces of oxygen in the atomizer atmosphere is required for hydride atomization. Hydrogen is usually present since it is formed by decomposition of tetrahydroborate used for the chemical generation of hydrides [1]. Oxygen demand is usually covered by oxygen contaminating sample, reagent solutions and gases [2]. Alternatively, a very small oxygen flow rate is introduced to the atomizer optical tube by a special capillary.
(ii) The most often employed miniature flame device is miniature diffusion flame (MDF). It is very simple - just a vertical quartz tube (support tube) serving as the burner of the argon/hydrogen mixture introduced to the atomizer together with the analyte hydride [3].
To make use of the full potential of analytical procedures for trace elemental and speciation analysis by AAS and AFS based on generation of VS the atomization step has to be optimized. Such an optimization should eventually lead to a hydride atomizer providing most notably [4] (a) in combination with the chosen detector (AAS or AFS) signal-to-noise ratio as high as possible to reach extremely low detection limits and (b) resistance to atomization interferences. This is our long-term goal.
Common atomizers of volatile species in AAS and AFS
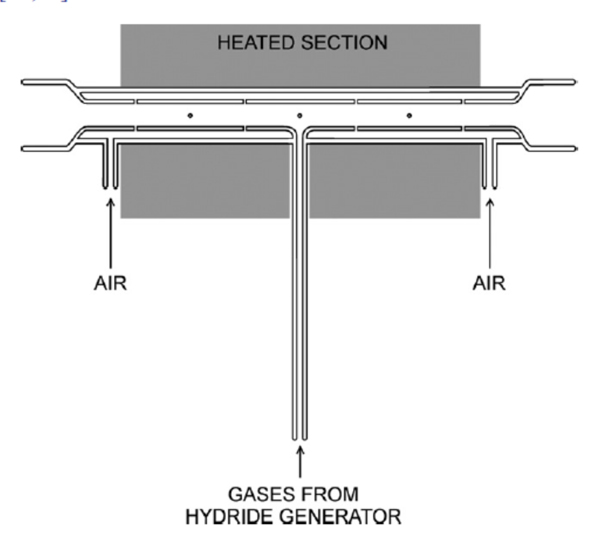
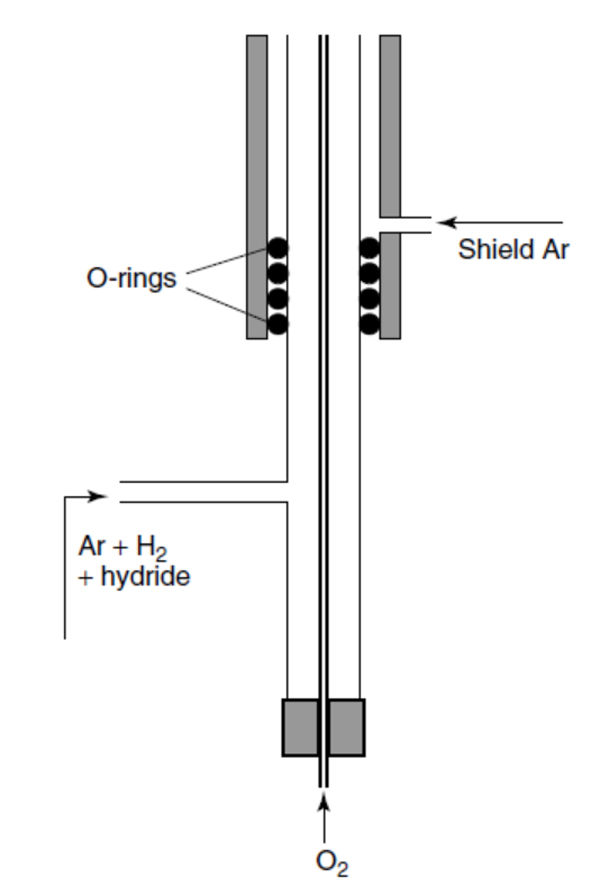
We investigated both types of atomizes of VS. We concentrated to the most popular group of analytically useful volatile species - hydrides. The novel type of QTA developed by us, multiatomizer [5,6], has a similar design as the conventional QTA except there is a provision making possible to introduce microflows of oxygen at multiple points all over the surface of the optical tube – see Fig. 1. The multiatomizer overcomes principal disadvantage of conventional QTA - low resistance to atomization interferences. We also developed an advanced microflame device - flame-in-gas shield (FIGS) atomizer [4,7]. It makes use of an argon shielded, highly fuel rich hydrogen oxygen micro flame. FIGS is much more flexible than MDF, offers better sensitivity and, mainly, a much higher potential in terms of miniaturization [4]- see Fig. 2.
Validation of theory of hydride atomization– optimization of the atomization step
Our present efforts aim to validation of the theory. The validation is of the upmost importance since if the radical theory is correct, its straightforward outcome is that the best way to optimize the atomization step is to establish how the relevant operation parameters influence the distribution of H radicals. To validate the theory, determination of distributions of H radicals and of analyte free atoms is required.
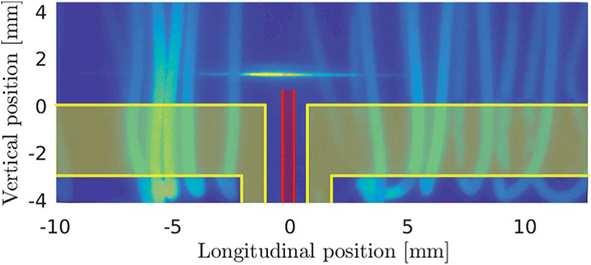
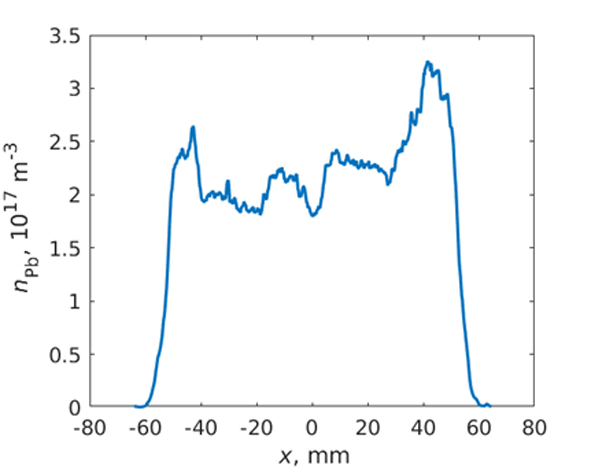
In principle, distribution of H radicals can be find out either experimentally or by a numerical simulation. Evidently, the numerical simulation is the simplest way providing that the output of the simulation is experimentally verified. Regarding the experimental determination of H radical distribution, extremely complex techniques are required - it took over four decades after formulation of the radical theory to prove conclusively the existence of H radical population in a QTA: very recently, we employed two photon absorption laser induced fluorescence (TALI-AFS) to determine H radical distribution in the conventional QTA [15]. H radicals are concentrated in a very small cloud in the center of the optical arm and their peak concentration exceeds four orders of magnitude the typical analytical concentration of hydride [15] - see Fig. 3. Using TALI-AFS, we were also the first to quantify the distribution of H radicals in DBD [16] and in MDF [17].
The other breakthrough we accomplished was a successful numerical simulation of H radical distribution in MDF atomizer based on computational model we built also very recently [17] after several unsatisfactory attempts. The model predicting distributions of H radicals and temperature for arbitrary combination of inlet gas flow rates to MDF atomizer was validated by a comparison with distributions determined by TALI-AFS [17].
A quantification of free atom distribution of an analyte in a hydride atomizer has not been reported in the literature before the end of 2019. However, our very recent observations proved that LI-AFS can be employed to quantify the distribution of free atoms at analytical concentrations in various hydride atomizers. In 2020 we published distributions of Pb in conventional QTA (see Fig. 4) and in DBD [18] and distributions of Te in DBD [19].
References
- [1] J. Dědina, D.L. Tsalev, Hydride Generation Atomic Absorption Spectrometry, John Wiley & Sons, Inc., Chichester, 1995.
- [2] A. D'Ulivo, J. Dědina, L. Lampugnani, Effect of contamination by oxygen at trace level in miniature flame hydride atomizers, Journal of Analytical Atomic Spectrometry, 20 (2005) 40-45.
- [3] J. Dědina, A. D'Ulivo, L. Lampugnani, T. Matoušek, R. Zamboni, Selenium hydride atomization, fate of free atoms and spectroscopic temperature in miniature diffusion flame atomizer, Spectrochim. Acta Pt. B-At. Spec, 53 (1998) 1777-1790.
- [4] J. Dědina, Atomization of volatile compounds for atomic absorption and atomic fluorescence spectrometry: On the way towards the ideal atomizer, Spectrochim. Acta Part B, 62 (2007) 846-872.
- [5] J. Dědina, T. Matoušek, Multiple microflame - a new approach to hydride atomization for atomic absorption spectrometry, J. Anal. At. Spectrom, 15 (2000) 301-304.
- [6] T. Matoušek, J. Dědina, A. Selecká, Multiple microflame quartz tube atomizer - further development towards the ideal hydride atomizer for atomic absorption spectrometry, Spectrochim. Acta Pt. B-At. Spec, 57 (2002) 451-462.
- [7] J. Dědina, A. D'Ulivo, Argon shielded, highly fuel-rich, hydrogen-oxygen diffusion micro flame - a new hydride atomizer, Spectrochim. Acta Pt. B-At. Spec, 52 (1997) 1737-1746.
- [8] J. Dědina, I. Rubeška, Hydride atomization in a cool hydrogen-oxygen flame burning in a quartz tube atomizer, Spectrochimica Acta Part B-Atomic Spectroscopy, 35 (1980) 119-128.
- [9] B. Welz, M. Melcher, Mutual interaction of elements in the hydride technique in atomic absorption spectrometry. Part 1. Influence of selenium on arsenic determination, Analytica Chimica Acta, 131 (1981) 17-25.
- [10] B. Welz, M. Melcher, Investigations on atomization mechanisms of volatile hydride forming elements in a heated quartz cell heated quartz cell. Part 1. Gas-phase and surface effects; decomposition of arsine, Analyst, 108 (1983) 213-224.
- [11] B. Welz, M. Schubert-Jacobs, D.L. Styris, D.A. Redfield, Investigation of reactions and atomization of AsH3 in a heated QT using AAS and MS, Spectrochimica Acta Part B-Atomic Spectroscopy, 45 (1990) 1235-1256.
- [12] J. Dědina, Quartz tube atomizers for hydride generation atomic absorption spectrometry : mechanism of selenium hydride atomization and fate of free atoms, Spectrochimica Acta Part B-Atomic Spectroscopy, 47 (1992) 689-700.
- [13] P. Stauss, Untersuchungen zu den reaktionen in der quartzkuvette der hydrid- technik der atomabsorptionsspektrometrie, dissertation, in, Fakultat fur Chemie der Universitat Konstanz, Konstanz, 1993, pp. 149.
- [14] B. Welz, M. Sperling, Atomic Absorption Spectrometry, 3 ed., Wiley-VCH, Weinheim, 1999.
- [15] P. Dvořák, M. Talába, J. Kratzer, J. Dědina, Radical theory of hydride atomization confirmed after four decades – determination of H radicals in the quartz hydride atomizer by two-photon absorption laser-induced fluorescence, Chemical Science, (2019) 3643–3648.
- [16] P. Dvořák, M. Talába, A. Obrusník, J. Kratzer, J. Dědina, Concentration of atomic hydrogen in a dielectric barrier discharge measured by two-photon absorption fluorescence, Plasma Sources Science and Technology, 26 (2017) 085002 (085013pp).
- [17] A. Obrusník, P. Dvořák, J. Dědina, An open-source tool for predictive simulation of diffusion flame atomizers in analytical chemistry, J. Anal. At. Spectrom., 35 (2020) 1464-1471.
- [18] M. Albrecht, M. Mrkvičková, M. Svoboda, J. Hranicek, J. Voráč, P. Dvořák, J. Dědina, J. Kratzer, Atomization of lead hydride in a dielectric barrier discharge atomizer: optimized for atomic absorption spectrometry and studied by laser induced fluorescence Spectrochim. Acta Part B, 166 (2020) 105819.
- [19] K. Bufková, S. Musil, J. Kratzer, P. Dvořák, J. Dědina, Hydride generation of tellurium and atomization in dielectric barrier discharge atomizer for atomic absorption spectrometry, Spectrochim. Acta Part B, (2020).